Generation of TREK-2 nanobodies
Nanobodies were produced as part of the Nanobodies4Instruct program in a method described by Pardon et al. 31. Briefly, purified human TREK-2 protein, identical to that used in previous structural studies9 was used to elicit an immune response in llamas. The resulting immune repertoire was isolated and positive clones were selected by phage panning and screening against the same TREK-2 protein. Overall, a panel of 29 unique nanobodies were produced (Supplementary Fig. S1) and these were further examined for their ability to produce a peak shift on size exclusion chromatography (SEC) to determine which nanobodies were capable of forming tight complexes (Fig. 1A). These tight binders were considered more suitable for subsequent structural and/or functional applications.
A Whole-cell currents recorded (at +40âmV) from oocytes expressing TREK-2 in the presence of a panel of nanobodies generated against TREK-2. Currents in the presence of extracellular nanobody (0.5âµM) were normalised to those in its absence. A threshold of 50% activation or inhibition was set in this screen (dotted lines). Tight binders within this panel were identified on the basis of producing a shift in size exclusion chromatography (SEC-shift) and shown in red. Asterisks mark the functionally active nanobodies plus an inactive tight binder chosen for further investigation. (Error bars represent meanâ±âS.D; nââ¥â5) B Crystal structures of the three functionally active nanobodies in complex with TREK-2. The nanobodies are shown in purple as surface representations whilst TREK-2 is shown in cartoon (green and gold). For CA10767 (Nb-Activator-67) the third âintracellularâ nanobody in the unit cell is shown in grey. A structure of the functionally inactive binder, CA10758 (Nb-Binder-58) is also shown in complex with TREK-2. In this case the nanobody binds as a dimer to the top of the Cap domain away from the extracellular TM/Pore loops that influence channel gating.
Screening for functional effects
To determine the possible functional effects of the nanobodies on channel activity, human TREK-2 channels were expressed in Xenopus oocytes and currents measured before and after the extracellular addition of nanobody (0.5âμM). An increase (or decrease) in the current size by more than 50% was used as the initial threshold in this screen. The results are shown in Fig. 1A and reveal that from this initial panel, three exhibited clear functional modulation of TREK-2: nanobody CA10761 inhibited channel activity, whilst CA10767 and CA10776 both activated TREK-2. Functional effects of these nanobodies were also consistent with their tight binding as determined in the SEC-shift assay (Fig. 1A). These modulatory nanobodies were then selected for further structural studies. Another tight binder that exhibited no functional effect (CA10758) was also included.
Nanobody binding to the extracellular Cap domain
Structures of these four nanobodies in complex with TREK-2 were then determined by X-ray crystallography using approaches similar to those previously described4,9 and were resolved between 2.4âà and 3.6âà resolution. For clarity, these nanobodies were also renamed to reflect their functional effect (Fig. 1B and Supplementary Fig. S2 and Table S1).
All four of these nanobodies exhibited binding to the extracellular Cap domain of TREK-2 (Fig. 1B). Interestingly, the functionally inactive binder only interacted with the extracellular tip of the Cap domain whilst the modulatory nanobodies interact with both the Cap and extracellular loops much closer to the selectivity filter where the primary gate is located. In one case (CA10767), binding was also observed to the intracellular face as well as the Cap domain. The properties of these structures and the functional effects of each modulatory nanobody are described in more detail below.
Immunodetection of TREK-2 expression at the cell surface
Previous studies have demonstrated the usefulness of nanobodies for immunodetection as well as in various immunoassays and other nanobody-based protein targeting strategies20,29. The structures determined above show that these TREK-2 nanobodies bind to the extracellular Cap domain indicating that immunodetection of TREK-2 at the cell surface should be possible. To examine this, we chose the functionally inactive nanobody CA10758 which interacts with the most exposed tip of the Cap domain. This nanobody is hereafter referred to as Nb-Binder-58 (or Nb58).
Many K2P channels exhibit N-linked glycosylation to their extracellular surfaces that may affect nanobody binding32. However, only one glycosylation site exists within TREK-2 in the extracellular loop that precedes Pore-Helix-1 (PH1). None of the four chosen nanobodies interact with or near this loop. Instead, Nb-Binder-58 binds as a dimer to the very tip of the Cap domain where it interacts with several residues that are unique between TREK-2 and the closely related TREK-1 channel (Fig. 2A).
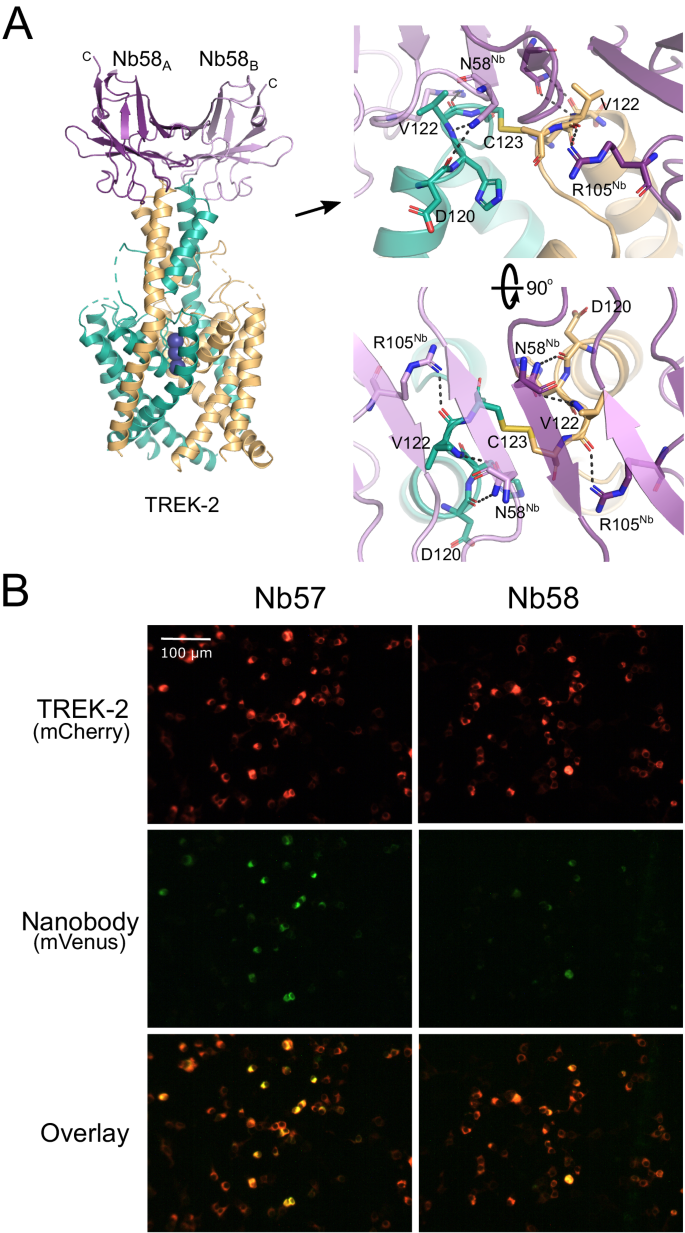
A Crystal structure of Nb-binder-58 (Nb58) in complex with TREK-2. This functionally inactive tight binder interacts as a dimer with the tip of the Cap domain only. The relative position of the C terminus of each Nb58 chain is indicated. The right-hand panel shows an expanded view of the interactions involved and shows the C123 disulphide between chains at the apex of the Cap. D120 and V122 are unique in TREK-2 compared to other TREK channels. B Immunodetection of TREK-2 at the cell surface. TREK-2-mCherry fusions were expressed in HEK-293 cells and stained with either Nb58 (or the highly related Nb57) fused to mVenus via the C-terminus indicated in panel A. Overlay of fluorescent signals shows clear binding at the extracellular surface. No overlapping signals are seen in untransfected controls or with other K2Ps (see Supplementary Fig. S3). These results were repeatable in nââ¥â3 independent replicates.
To determine whether this nanobody can detect TREK-2 expression at the cell surface, Nb-Binder-58 was fused to the mVenus fluorescent protein and applied to HEK-293 cells expressing TREK-2 tagged with the mCherry fluorescent protein. Figure 2B shows overlapping signals that are not observed on cells expressing TREK-1, nor for any other K2P channel (Supplementary Fig. S3). A similar, functionally inactive nanobody (CA10757: Nb-Binder-57 or Nb57) that exhibits almost complete sequence identity with Nb-Binder-58 is also capable of similar immunodetection of TREK-2 (Fig. 2B and Supplementary Figs. S1 and S3). This indicates that both these nanobodies represent useful tools for the specific immunodetection of homomeric TREK-2 channels.
A partially-selective activatory nanobody
The first activatory nanobody (CA10767) hereafter referred to as Nb-Activator-67 (or Nb67), binds to the side of the Cap domain (Fig. 3A) with all three CDR loops interacting with the external face of the Cap helices. However, these interactions are not symmetrical and on one side the EH1 Cap helix is partially unwound at the midpoint (residues 107â109; Fig. 3C). The structure also reveals a nanobody bound to the intracellular side where it occludes the entrance to the inner cavity (Fig. 3A). However, upon closer examination of the crystal structure this âintracellularâ interaction appears to result from tetramerisation of the nanobody within the unit cell that may drive the crystal packing and the resulting non-canonical structure (Supplementary Fig. S4A). Nevertheless, to rule out any functional effects of this intracellular interaction we examined the effect of this nanobody on TREK-2 currents in excised patches where it could be applied to the intracellular surface of the membrane. No functional effect on TREK-2 activity was observed (Supplementary Fig. S4B). This is in marked contrast to its clear activatory effect when applied extracellularly (Fig. 1A). Further examination revealed that Nb-Activator-67 rapidly increased channel activity ~3â4 fold with nanomolar efficacy (EC50â=â101â±â12ânM) when perfused onto oocytes expressing human TREK-2 (Fig. 3B).
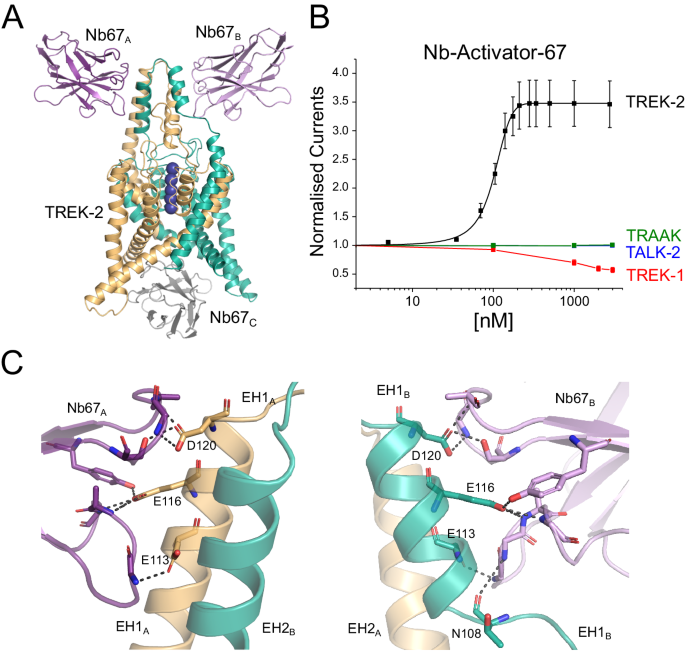
A Crystal structure of TREK-2 in complex with Nb-Activator-67 (Nb67). Nanobodies bind to each side of the Cap domain and on one side (green subunit) the Cap helix unwinds. A third Nb67 (grey) is also found in the asymmetric unit bound to the intracellular surface. These unusual features may arise from the tetramerisation of Nb67 within the crystal (see Supplementary Fig. 4A). B Dose-response relationships showing extracellular activation of TREK-2 channel activity by Nb67. Whole-cell TREK-2 currents were recorded at +40âmV and perfused with different concentrations of Nb67. Currents were normalised to those recorded prior to the application of nanobody. No activation of TRAAK or TALK-2 K2P channels was observed, but for the related TREK-1 up to 50% inhibition of channel activity was seen at concentrations of Nb67â>â1âµM. (Error bars represent meanâ±âS.D; nââ¥â3). C Expanded view of the interaction of Nb67 with the two sides of the Cap domain. The interaction with Nb67A (Left) shows no distortion of the helices, whereas interaction with N67B on the opposite side results in unwinding of EH1B (residues E113, E116 and D120 are unique in TREK-2 vs TREK-1). Right: the interaction of N108 with NB67B differs on the side where EH1B is unwound. This interaction does not occur with Nb67A.
To address its selectivity, we examined effects on other K2P channels. No functional modulation was observed on either TALK-2 or TRAAK. However, an inhibitory effect was observed on the closely related TREK-1 channel at high concentrations of nanobody >1âμM (Fig. 3B). This opposite effect initially appeared interesting because of the opposite effects of extracellular pH on the activity of TREK-2 and TREK-1, and the role of the extracellular Pore/TM loops in this process33. However, Nb-Activator-67 does not appear to contact these loops and instead only interacts with the extracellular face of the Cap domain resulting in an unwinding of the EH1 Cap helix on one side (Fig. 3C). Intriguingly, both M4 helices in this TREK-2 structure are in the Up conformation consistent with a more activated state of the channel (Fig. 3A), although this could also be due to its interactions with the nanobody bound to its intracellular surface. Full occupancy of ion binding sites within the filter of TREK channels is also usually associated with an activated state of the channel9,34, and in this structure all the sites appear occupied, albeit in an asymmetric fashion due to unwinding of the Cap on one side (Fig. 3A). Although it is tempting to associate the effect of unwinding of EH1 with the activatory effects of Nb-Activator-67, the crystal packing interactions make interpretation of this particular structure challenging. Due to the additional lack of specificity between TREK-1 and TREK-2, the mechanism of activation by this nanobody was not investigated further. However, its robust activatory effect on recombinant homomeric TREK-2 channels, especially at lower concentrations, indicates that this nanobody may remain a useful tool in situations where the identity of its target is already known.
A selective activatory nanobody
Unlike Nb-Activator-67, the second activatory nanobody (CA10776, hereafter referred to as Nb-Activator-76 or Nb76) exhibited highly selective activation of TREK-2 with an efficacy of 412â±â14ânM and had no effect on TREK-1 or other K2P channels tested, even when used at high concentration (Fig. 4A & Supplementary Fig. S4C). The structure of this nanobody in complex with TREK-2 reveals extensive contacts between the side of the Cap domain and the CDR loops (Fig. 4B). Interestingly, this involves many residues at the intersubunit interface, several of which are unique in TREK-2 including E113, H135 and Q127. The most significant contact involves W98 on CDR3 of the nanobody which inserts into the intersubunit groove between helices in the Cap domain to form a polar interaction with E128 of TREK-2. Also, R53 on CDR2 helps anchor the nanobody to the Cap helices by H-bonding with Q106 on TREK-2 (Fig. 4C). The importance of these two interactions is supported by the fact mutation of either of these residues on Nb-Activator-76 (W98A or R53A) severely impaired its activatory effect (Fig. 4A), but whether they disrupt binding or nanobody efficacy remains to be determined.
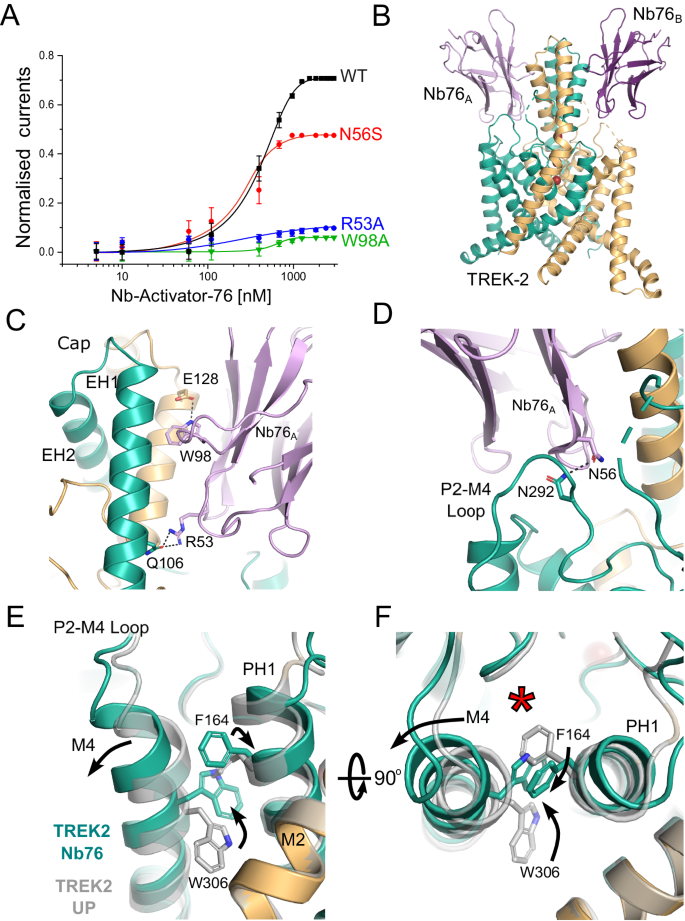
A Dose-response relationships showing activation of TREK-2 channel activity by Nb-Activator-76 (Nb76). Whole-cell TREK-2 currents were recorded at +40âmV and perfused with different concentrations of Nb76. The effect of mutating different residues in Nb76, which interact with TREK-2, are shown. The curves shown for the R53A and W98A mutant nanobodies were fitted by hand. (Error bars represent meanâ±âS.D; nââ¥â5). B Crystal structure of TREK-2 in complex with Nb76 bound to both sides of the Cap and the P2-M4 loop. C Interactions with the side of the Cap. W98 on CDR3 inserts between Cap helices of different TREK-2 subunits to form an interaction with E128 on EH2 of TREK-2, whilst R53 on Nb76 interacts with Q106 on EH1 of a different subunit of TREK-2. Mutation of either W98 or R53 on Nb76 reduces its activatory effect. D Expanded view of the interaction of N56 on CDR3 with N292 on the P2-M4 loop. Mutation of N56 also reduces activation by Nb76. E Alignment with TREK-2 Up-state (4BW5 in Grey) highlights displacement of the top of M4 and the inward rotation of W306. This is accompanied by the outward rotation of F164 on Pore-Helix-1 (PH1). F The right-hand panel rotates this view through 90° to better visualise the relative reorientation of W306 and F164 and the outward displacement of M4. This expands the K2P modulator site behind the selectivity filter (red asterisk).
However, the most important functional interactions likely involve contacts with the extracellular P2-M4 loop of TREK-2 that has been implicated in the regulation of filter-gating33. In particular, N56 on CDR2 H-bonds with N292 on the P2-M4 loop of TREK-2 (Fig. 4D). The importance of this interaction with the P2-M4 loop is confirmed by mutation of N56 on Nb-Activator-76 which reduces its activatory effect, (Fig. 4A). Consistent with an activated state, both M4 helices in this structure are also in the Up conformation (Fig. 4F). The role of ions in gating the filter is unclear because crystals of sufficient quality could only be obtained using a crystallisation solution containing Ba2+ which contributes to the crystal contacts. However, this does not change the selectivity filter in comparison to the Up-state conformation (PDB ID 4BW5).
ML335 is a small molecule TREK channel agonist that binds within the âK2P Modulator Pocketâ, a cryptic binding site created by the displacement of the top of M4 where its interactions with the P2-M4 loop and Pore-Helix-1 (PH1) are proposed to stabilise TREK-2 in a more conductive conformation11,17,35. It is therefore highly significant that the interaction of Nb-Activator-76 with the P2-M4 loop also induces a displacement in the top of the M4 helix similar to that seen in the ML335-bound structures of TREK-2 (Fig. 4E). Furthermore, it has been reported that the reorientation of a tryptophan residue at the top of M4 (W306 in TREK-2) also correlates with the activated state of TREK channels8,10,12 and that any hydrophobic moiety occupying this space is capable of stabilising this activated state10. In this nanobody bound structure W306 side chains in both helices are in the inward orientation and occupy this space. But unlike other TREK structures where this M4 tryptophan has rotated inwards, here the adjacent residues on PH1 (F164) also flips orientation to interact with the top of M4 to stabilise this displacement (Fig. 4F). This demonstrates a mechanism whereby movement of the M4 helix may communicate with PH1 to influence the selectivity filter gate and highlights the importance of this âK2P modulator pocketâ in channel gating36.
An inhibitory nanobody with a dual mechanism of action
The inhibitory nanobody, CA10761, hereafter referred to as Nb-Inhibitor-61 (or Nb61), rapidly inhibited TREK-2 currents (IC50â=â685â±â18ânM; Fig. 5A). This inhibitory effect appears specific as this nanobody has no functional effect on the related TREK-1 or on TASK-3 channels but is active against mouse TREK-2 (Supplementary Fig. S5A). The structure of the nanobody/TREK-2 complex indicates only one nanobody bound per asymmetric unit, but this appears to result from its crystal packing where interactions between the Cap domains prevent binding of a second nanobody to the opposite side (Fig. 5B).
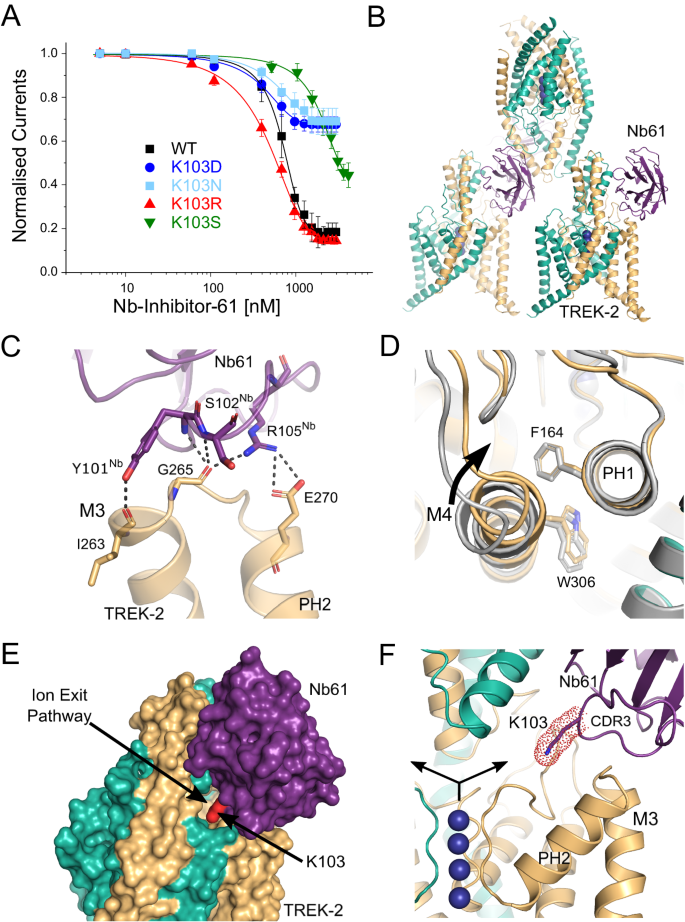
A Dose-response relationships showing inhibition of TREK-2 channel activity by Nb-Inhibitor-61 (Nb61). Whole-cell TREK-2 currents were recorded at +40âmV and perfused with different concentrations of Nb67. Also shown are the effect of different mutations of K103 in Nb61. (Error bars represent meanâ±âS.D; nââ¥â5). B The crystal structure unit cell reveals only one Nb61 bound to TREK-2; packing interactions between the Cap domains within the crystal prevent binding to the other side. C Expanded view of the major interactions of Nb61 with the top of M3 and the M3-PH2 loop. D Alignment with TREK-2 Up-state (4BW5 in Grey) highlights displacement of M4 and PH2-M4 loop inwards towards the K2P modulator pocket. E Surface view showing obstruction of the extracellular ion exit pathway by Nb61 (in purple). K103 within the obstructed pathway is also marked (in red). F Expanded view of the location of K103 within the ion exit pathway. K+ ions are shown as purple spheres and the arrows indicate the direction of K+ flow through the ion exit pathway that is obstructed by K103 on CDR3 of Nb61.
The position of Nb-Inhibitor-61 on the side of the Cap domain allows it to form extensive interactions between the Cap helices and CDR1, as well as with the β5 sheet and β5-β6 loop. In addition, its long CDR3 loop forms multiple interactions with the top of M3 and the M3-PH2 loop (Fig. 5C) as well as additional interactions with the top of M2 and the P2-M4 loop. However, the most striking feature of this long CDR3 loop is that it also inserts a lysine residue (K103) deep within the extracellular ion exit pathway that emerges just below the Cap domain (Fig. 5E, F). Overall, the position of this nanobody obstructs most of this ion exit pathway with the remaining gap being only 1.35âà wide compared to >4âà in the apo state. Intriguingly, the position of this positively charged lysine within the ion exit pathway is reminiscent of the way some toxins block K+ channels via a âcork in a bottleâ or âplugâ type mechanism37. It also shares similarities with the âfinger in the damâ mechanism of TREK channel block by Ruthenium Red where interaction of this positively charged inhibitor with the âkeystone inhibitor siteâ below the Cap domain directly blocks K+ permeation7,36,38. Unlike these other toxins, K103 does not insert far enough into the pathway to interact directly with ions exiting the filter, but it clearly has the ability to impede K+ movement through this exit pathway and so we examined its role further.
A critical role for Lysine 103 in channel inhibition
This lysine appears to make no specific H-bonding or charged interactions within the ion exit pathway and mutation to a serine (K103S) resulted in a marked reduction in its inhibitory effect (Fig. 5A). We also examined other mutations at this position (K103R, K103N and K103D). Consistent with a role for a positively charged residue at this position, the K103R mutant produced similar, if not greater, inhibition whilst neutral or negative substitutions (K103N and K103D) resulted in a markedly reduced inhibitory effect (Fig. 5A). We also examined the effect of this nanobody on TREK-2 single channel activity (Supplementary Fig. S5B). Due to the low intrinsic open probability of TREK-2, only a qualitative comparison was made between the behaviour of multi-channel patches with or without Nb-Inhibitor-61 included in the pipette solution. This revealed a marked suppression of TREK-2 activity via reduction in the durations of bursts of openings and increase in the time spent in the long-closed states. This is consistent with the idea this nanobody acts as a slow blocker resulting in a complete cessation of K+ flux through the channel.
Possible allosteric effects of Nb-Inhibitor-61
Together, these results with Nb-Inhibitor-61 support the contribution of a direct toxin-like (or âfinger in the damâ) block of ion permeation by the nanobody38. However, the bifurcation of the ion exit pathway means that such a mechanism would also require nanobody binding to both sides of the Cap domain. There are no obstructions to the binding of a second nanobody on the other side, but due to crystal packing, only one is observed in this structure. It is therefore possible that other, allosteric mechanisms may also contribute to its inhibitory properties. For example, multiple effects are also seen in some toxins where, in addition to direct pore block, interactions with the external pore loops result in allosteric modulation of channel gating39,40. For Nb-Inhibitor-61, this may arise through the extensive contacts it makes with the M3-PH2 loop as well as with the P2-M4 loop and M2/M3 helices. Of particular note are the interactions with both I263 and G265 at the top of M3 (Fig. 5C) because E264 has been shown to play an important role in the regulation of the filter gate in TREK channels, thus interaction with the two adjacent residues might easily influence this gating mechanism11.
Consistent with such an allosteric effect, the M4 helix of the subunit that interacts with this nanobody is in the Down conformation (Fig. 5B). Furthermore, the relative orientation of side chains for both W306 on M4 and F164 on PH1 are reversed compared to their positions the Nb76/TREK-2 structure and its interaction with the P2-M4 loop pulls the top of M4 closer to PH1 compared to the activated state induced by Nb-Activator-76 (Fig. 5D). However, all 4 ion binding sites in the filter appear to be occupied and no obvious structural changes are seen in the filter itself or in the surrounding region. Thus, although additional allosteric effects of this nanobody on the filter gate cannot be excluded, Lysine 103 clearly contributes to channel inhibition by directly blocking the ion exit pathway.
A biparatopic inhibitory nanobody with improved sensitivity
The two activatory nanobodies identified in this study function with relatively high efficacy (EC50~100 and 300ânM) compared to Nb-Inhibitor-61 (IC50~650ânM). Multivalency is a well-established method for increasing the affinity of ligands, and linked biparatopic nanobodies which target spatially discrete epitopes have also proven an effective way of increasing their functional efficacy41,42,43.
We therefore attempted to increase the sensitivity of Nb-Inhibitor-61 by linking it to the non-functional Cap binder (Nb-Binder-58). Comparison of the TREK-2/Nanobody structures (Fig. 1B) revealed non-overlapping binding sites for these two nanobodies with each nanobody separated ~21âà between the C-terminus of Nb-Inhibitor-61 and the N-terminus of Nb-Binder-58. The two nanobodies were therefore linked in frame using a (Gly-Ser)4 linker to create a divalent biparatopic nanobody we refer to as Nb-Inhibitor-6158 (or Nb6158).
Analysis of the relative binding affinity of this biparatopic nanobody using bilayer interferometry revealed a marked increase in affinity for TREK-2 of at least 250-fold (4.1â±â0.1ânM, nâ=â4) compared to monomeric Nb-inhibitor-61 (1100â±â30ânM, nâ=â4;). Nb6158 also exhibited a 16-fold increase in its inhibitory efficacy from 651â±â18ânM to 40â±â3ânM, nâ=â5 (Fig. 6A). This demonstrates the suitability of linking nanobodies in âdaisy-chainâ arrangements to modulate their binding properties.
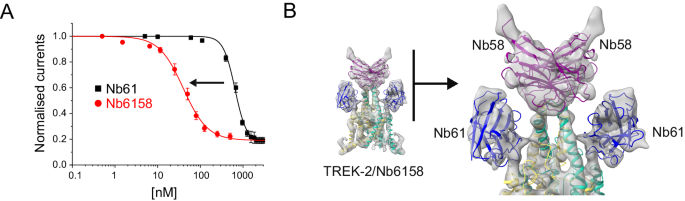
A Dose-response relationships showing inhibition of TREK-2 channel activity by the divalent linked Nb-Inhibitor-6158 (Nb61). Whole-cell TREK-2 currents were recorded at +40âmV and perfused with different concentrations Nb6158. Consistent with its markedly increased affinity, this linked nanobody exhibits a marked increase in its inhibitory efficacy compared to Nb61 alone. (Error bars represent meanâ±âS.D; nââ¥â5) B A 7.4âà resolution CryoEM structure of TREK-2 in complex with Nb6158 shows two copies of Nb61 bound, one to each side of the Cap domain. An expanded view of their relative position is shown on the right. This indicates that, in addition to any allosteric effects, Nb61 is capable of interacting with TREK-2 on both sides of the Cap domain to obstruct K+ permeation from both extracellular ion exit pathways.
The structures above show that Nb-Binder-58 binds as a dimer to the Cap domain suggesting that two copies of Nb-Inhibitor-61 in this biparatropic nanobody may now be able to bind more efficiently and directly occlude both ion exit pathways. Given the arrangement of the unit cell in the crystal structure of the TREK-2/Nb61 complex which only shows one Nb61 bound, we attempted to determine its structure in complex with TREK-2 by cryo-electron microscopy (Fig. 6B and Supplementary Fig. S6). At ~7.4âà resolution, the map was sufficient to reveal two copies of Nb-Inhibitor-6158 bound with one Nb-Inhibitor-61 domain on either side of the Cap domain in an identical position to that seen in the crystal structure. This confirms that this nanobody can interact with both subunits of TREK-2 and potentially occlude both extracellular ion exit pathways.
In this study, we describe the generation and characterisation of a panel of nanobody binders to the TREK-2 K2P channel resulting in one inhibitor, two activators and other nanobodies suitable for immunodetection of TREK-2. One activator in particular (Nb-Activator-76) elicits highly selective activation of TREK-2. These nanobodies therefore not only provide useful probes for analysing the structure and function of homomeric TREK-2 channels but also identify a class of highly specific extracellular modulators that can be further investigated for therapeutic potential.
Ever since the first structures of K2P channels were resolved over a decade ago the role of their large extracellular Cap domain has remained unclear34,44. It was initially suggested this domain may have evolved to protect the selectivity filter of K2P channels from the small peptide toxins that often target other members of the superfamily of tetrameric cation channels. However, the complex domain swap involving the M1 helix required to assemble this extracellular domain suggests this is an unlikely strategy, especially as examples of toxin resistance in ion channels often only require single mutations in the extracellular toxin binding site45.
Nevertheless, the Cap domain is a defining feature of K2P channels, and whatever its purpose, it obviously makes the toxin block of the pore much less likely. It is therefore significant that we have identified a nanobody in which the longer CDR3 loop inserts a lysine sidechain directly into the extracellular ion exit pathway in a manner analogous to some K+ channel toxins. In the case of TREK channel inhibition by Ruthenium Red, this small positively charged inhibitor is able to access deeper into the exit pathway to block permeation directly above the filter, whereas it may not be possible for this CDR3 loop to insert deep enough to access the keystone Inhibitor site, even if it were longer. Nevertheless, this inhibitory nanobody is highly effective and its mechanism of action likely involves both allosteric and direct blocking effects on permeation. Also, the structure of this TREK-2/nanobody complex, and those of the others solved in this study, reveal that the Cap domain is highly immunogenic and provides a good scaffold on which nanobodies can anchor themselves to influence TREK channel function. We also demonstrate that the Cap domain allows the design of biparatropic nanobodies with improved affinity and functional efficacy, and the nanomolar efficacy of this linked Nb-inhibitor-5861 provides a powerful analytical tool for probing TREK-2 function.
In the case of the two activators, the first (Nb-Activator-67) is a highly effective probe for the study of homomeric TREK-2 when the identity of the target is known, but uncertainties about this crystal structure make interpretations of its mechanism of action difficult. However, the second activator (Nb-Activator-76) provides important insights into the mechanism of TREK channel activation and demonstrate how the extracellular P2-M4 loop and rearrangements at the top of the M4 helix play a critical role in the regulation of channel gating. Overall, these rearrangements expand current models for TREK channel gating via the K2P modulator site to suggest that outward displacement of the top of M4 changes the contacts between M4, the P2-M4 loop and Pore-Helix-1 to activate the selectivity filter gate, whereas displacement in the other direction results in the opposite effect and inhibition. Similar to that proposed for other TREK channel modulators8,10,17, this may also involve reorientation of the W306 and F164 side chains, or their equivalents (Fig. 7). This model is also consistent with recent observations that covalent attachment of ML335 derivatives within the K2P modulator site via a conserved serine on PH1 can irreversibly activate TREK channels35.
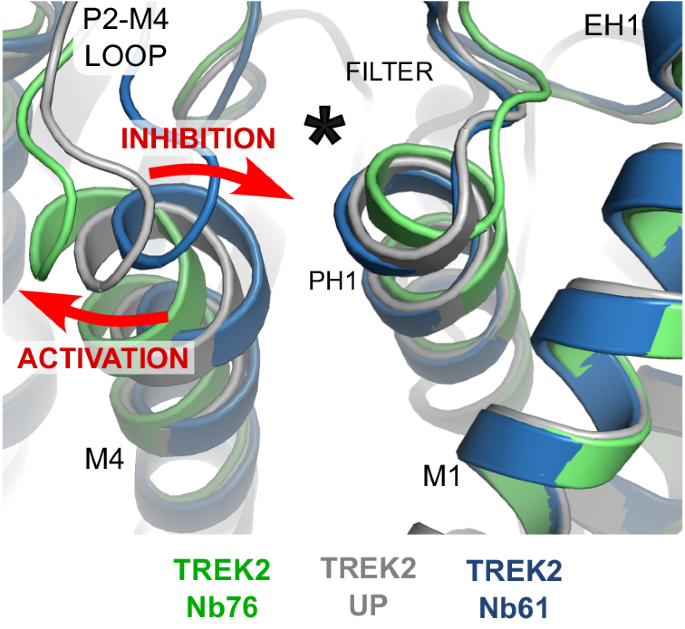
Alignment of the TREK-2/Nb76 and TREK-2/Nb61 complexes with the TREK-2 Up-state show that activation by Nb76 is associated with outward movement of the top of M4 and the P2-M4 loop whilst inhibition by Nb61 involves movement in the opposite direction (red arrows). The location of the K2P modulator site behind the selectivity filter is indicated by the asterisk.
However, the mechanism by which nanobody binding is then coupled to changes in ion occupancy within the filter and the dynamics of K+ permeation remain unclear. A limitation of this study is that the relative dynamics of these specific regions are probably critical for the regulation of gating, but most structures, including those in this study, are determined in high [K+] (>100âmM). This often results in high ion occupancy within the filter in such crystal structures, whereas the physiological situation is obviously very different and difficult to compare. It also remains to be determined how these modulatory nanobodies interact with other regulatory mechanisms such as temperature and stretch. However, the cooperative and polymodal nature TREK channel gating means any such interactions are likely to be complex and potentially difficult to dissect.
The nanobody âbindersâ that have no functional effects are also likely to be useful tools for the immunodetection of TREK-2, although future studies will be required to demonstrate the sensitivity required for detection in vivo. It is also known that TREK-2 can form heteromeric channels with either TREK-1 or TRAAK46,47, and nanobodies capable of detecting such combinations would be extremely powerful for dissecting the role of such heteromeric channels in vivo. It is therefore significant that the domain swap involved in the assembly of the Cap domain generates an intersubunit interface that forms extensive contacts with nanobodies in these structures and which would be unique in any heteromeric channel combinations; thus, if binders can differentiate between these interfaces in homomeric TREK channels, then it may be possible to generate similar nanobodies that are uniquely selective for heteromeric K2P channels.
Thus, although ion channels remain underexploited as antibody drug targets, this study demonstrates that the unique nature of the Cap domain combined with the role of the extracellular loops in K2P channel gating allows nanobodies to be considered as an exciting alternative to small molecules as highly selective probes to K2P channel structure-function studies, and of TREK channels in particular. This therefore expands the potential of nanobodies as effective therapeutics and their role in future drug development.