SOSG fluorescent staining of Candida
While using SOSG as a fluorescent probe to detect intracellular production of 1O2 by photosensitizers, a non-illuminated, heat-treated C. albicans control surprisingly stained positively with SOSG (Fig. 1a). No fluorescence was registered under the same conditions without heat treatment.
Fluorescent SOSG staining of heat-treated C. albicans. Two hundred µl aliquots of wild-type C. albicans (JYC5) cell suspensions were incubated in thermocycler at 95 °C for 5 min under (a) aerobic or (b) anaerobic atmosphere. The samples were cooled down, 10 µM SOSG was added to each sample, incubated 30 min in the dark, and images were captured by confocal fluorescent microscope.
It has been reported that exposure of Chlamydomonas to 40 °C resulted in fluorescent SOSG staining of the cells, attributed to generation of 1O2 by decomposition of lipid peroxides8. To exclude lipid peroxides-derived 1O2, the experiment was repeated in anaerobic Coy chamber. Heating the cells and incubation with SOSG was performed under anaerobic conditions. Results (Fig. 1b) demonstrate that treatment under anaerobic conditions did not prevent the fluorescent staining of the cells. Similar results were obtained with S. cerevisiae.
Adding SOSG 24 h after the heat treatment also resulted in fluorescent staining of the cells (Suppl. Fig. 1), which again points that it is not dependent on reactive species with short life or to short-lasting chemical reactions initiated during heating.
Since SOSG has been marketed as cell impermeable and heat is known to permeabilize microbial cells21,22,23, a possible explanation for the observed fluorescent staining of the heat-treated cells could be facilitated penetration of the fluorescent probe inside the cells.
To test if other membrane-permeabilizing agents cause SOSG staining, C. albicans cell suspension was incubated with chlorhexidine, an antiseptic with known cell envelope permeabilizing activity24,25,26. Direct interaction between chlorhexidine and SOSG has been avoided by thoroughly washing the cells before the addition of SOSG. As seen by the green fluorescence, chlorhexidine preincubation resulted in SOSG staining of the cells (Fig. 2a). Mechanical membrane disruption by sonication yielded similar results, with cells showing positive staining by SOSG (Suppl. Fig. 2).

Effect of pretreatment with chlorhexidine on SOSG fluorescent staining of C. albicans. Candida cell suspension in PBS-glucose were incubated for 30 min with 100 µg/ml chlorhexidine under (a) aerobic or (b) anaerobic atmosphere. The cells were washed with PBS, resuspended in PBS-glucose, 10 µM SOSG was added, incubated 30 min in the dark and images were obtained by confocal fluorescent microscope.
To rule out participation of ROS in the SOSG staining, incubation with chlorhexidine and SOSG staining were repeated in anerobic atmosphere. Results (Fig. 2b) show that anaerobiosis did not prevent the fluorescent staining of the cells. The conclusion that SOSG fluorescence was ROS-independent was strengthened by the fact that incubation of C. albicans with H2O2 at concentrations up to 10% did not result in fluorescent SOSG staining (Suppl. Fig. 3a). In contrast, addition of SOSG to cells preincubated with 3% tert-butyl hydroperoxide resulted in fluorescent staining (Suppl. Fig. 3b). This result can be explained by differences in the mechanisms of hydrogen peroxide and tert-butyl hydroperoxide induced cell damage27. While H2O2 kills cells mainly by causing DNA damage27,28,29, tert-butyl hydroperoxide disrupts membrane function resulting in loss of cell content27.
Gollmer et al.9 have pointed out that a small amount of SOSG-EP present as a contaminant could lead to misleading results. The fluorescent SOSG staining of cells without illumination and under anaerobic conditions raised a suspicion that the SOSG vials have been exposed to radiation during transportation, which increases the fluorescence of the probe13 or to improper storage leading to accumulation of SOSG-EP. To test such a possibility, we analyzed two different batches of SOSG received 13 years apart, one stored according to manufacturer’s instructions at − 24°C in unopened vials, the other analyzed immediately upon receipt. Analyses by UV–Vis and fluorescent spectroscopy revealed almost identical absorbance and fluorescent spectra. No indication of excessive SOSG-EP accumulation was found in the SOSG probe after prolonged storage. The absorption (Suppl. Fig. 4A) and fluorescence (Suppl. Fig. 4B) spectra of the two batches of SOSG closely resembled the spectra reported by other investigators9,30. A time-dependent increase of the intensity of SOSG florescence was observed when exposed to singlet oxygen generated by Rose Bengal (singlet oxygen quantum yield, ΦΔ = 0.7631) (Suppl. Fig. 4B). These results confirm that the two batches of SOSG can be used for detection of photochemically generated singlet oxygen. Illumination of SOSG with polychromatic light (115 mW/cm2 at 420 nm) in the absence of a photosensitizer increased the intensity of fluorescence in accordance with previous reports9,30 (Suppl. Fig. 4C). The spectral analyses, however, indicated that the two SOSG commercial preparations do not fulfil the requirement for zero SOSG-EP content, stipulated by Gollmer et al.9.
Since photogenerated 1O2 increases the intensity of SOSG fluorescence, we tested the effect of illumination of two photosensitizers, a commercial Rose Bengal and a previously investigated Zn(II) meso-tetrakis(N-hexylpyridinium-4-yl) porphyrin (ZnTnHex-4-PyP), which is known to disperse in membranes32, on the fluorescent SOSG staining of Candida albicans. Results demonstrated that illumination of cells in the absence of a photosensitizer did not result in fluorescent SOSG staining (Fig. 3a). A small number of cells were stained when illumination with Rose Bengal was performed (Fig. 3b) while almost all ZnTnHex-4-PyP loaded cells displayed fluorescence (Fig. 3c). Such differences in SOSG staining can be attributed to differences in the properties and cellular uptake of the two photosensitizers. Rose Bengal is anionic and hydrophilic and accumulates very inefficiently within cells33,34. In contrast, ZnTnHex-4-PyP is cationic and amphiphilic, which allows rapid uptake and accumulation in cells, particularly in membranes32. Irrespective of having comparable singlet oxygen quantum yields35, these two photosensitizers did not produce similar SOSG staining of Candida albicans.
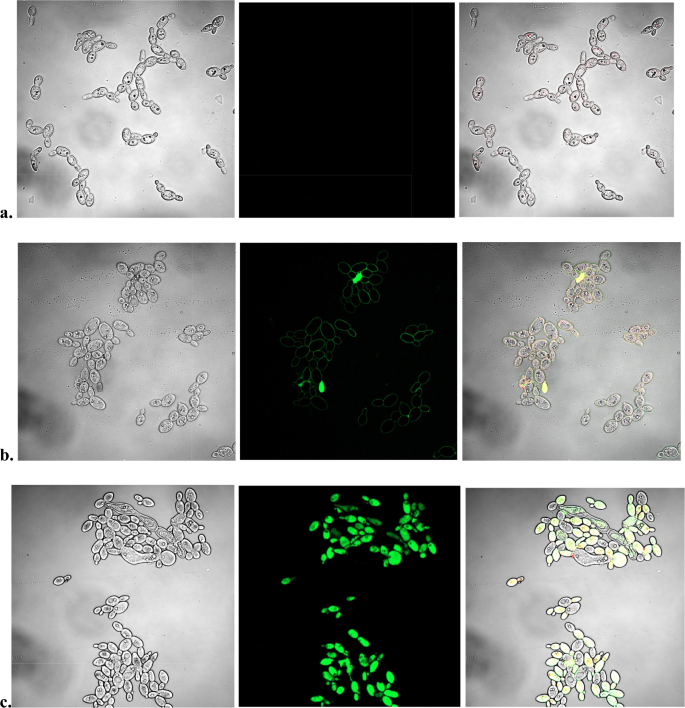
SOSG fluorescent staining of C. albicans illuminated in the presence of photosensitizers. Candida suspensions in PBS-glucose were incubated 30 min in the dark without a photosensitizer (a), with 20 µM Rose Bengal (b) or with 10 µM ZnTnHex-4-PyP (c). After the incubation, the cells were washed to remove the unbound photosensitizers and were resuspended to the same density in PBS-glucose. SOSG was added and samples were illuminated for 30 min. Rose Bengal containing samples were illuminated with green light (540–550 nm) at a fluence of 155 mW/cm2, and ZnTnHex-2-PyP loaded cells were illuminated with polychromatic light (136 mW/cm2 at 420 nm). Respective controls were illuminated with either with green or polychromatic light respectively. Panel (a) shows cells illuminated with green light. After the illumination images were captured using confocal fluorescent microscope.
At the same time, no obvious difference could be observed between samples stained after heating or chlorhexidine treatment, and samples exposed to photo-generated singlet oxygen.
These results suggest that fluorescent staining of cells as a result of various treatments reflects loss of membrane integrity rather than generation of singlet oxygen. To further explore such a possibility, SOSG fluorescent staining of bacterial and cancer cells was investigated.
SOSG fluorescent staining of bacteria
Incorporation of SOSG in bacterial cells is restricted by the presence of a complex cell wall and in Gram-negative bacteria, an outer membrane. To prevent binding of SOSG to components of the medium9, incubation of bacteria with SOSG was performed in PBS glucose. Incubation of E. coli cell suspensions in PBS-glucose without any other treatment, however, did not result in fluorescent SOSG staining of the cells (Suppl. Fig. 5a). Exposure of E. coli suspensions to temperatures below 60 °C also did not result in fluorescent staining irrespective of substantial loss of cell viability. The cells displayed green fluorescence only after incubated at 60 °C or higher temperature (Suppl. Fig. 5c,d). Heat-induced cell permeabilization was time dependent. The number of fluorescent cells increased by the increase of the time of incubation reaching a maximum at 30 min (Suppl. Fig. 6).
Sodium azide (NaN3) is well-known physical quencher of singlet oxygen and is commonly used to prove singlet oxygen involvement36,37. Addition of NaN3 up to 10 mM to E. coli cell suspension before heating, however, did not affect the fluorescent staining by SOSG (Fig. 4a).
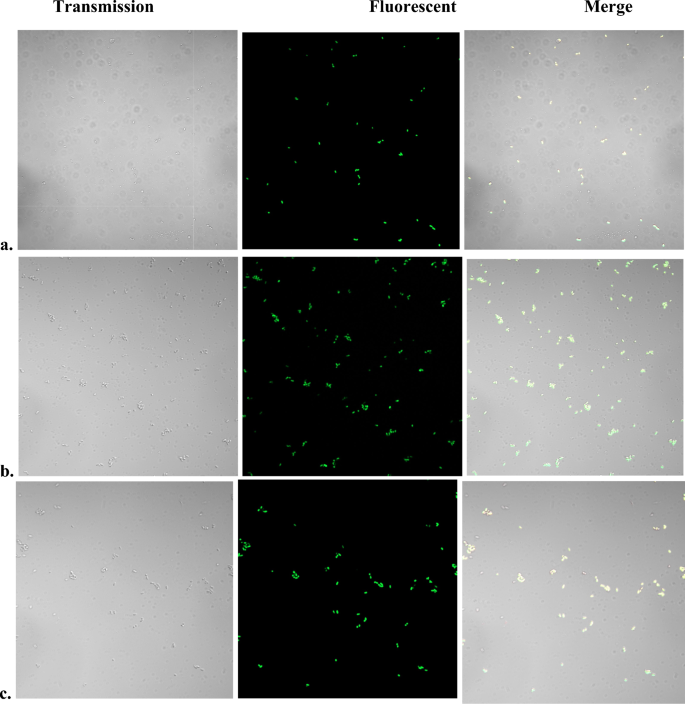
Fluorescent SOSG staining of heat-treated E. coli aerobically in the presence of azide or anaerobically. Stationary phase cultures grown in LB medium were washed with PBS and resuspended in PBS-glucose to OD600nm ~ 1.25. Aliquots were incubated for 15 min in a water bath at 95°–100° with or without NaN3. For anaerobic heat treatment, all manipulations were performed in a Coy anaerobic chamber and all solutions and samples were degassed. After the treatment, samples were cooled down and SOSG was added to a final concentration of 2.0 µM. Images were obtained 5–10 min. after the addition of SOSG. (a) Aerobically heat-treated control (no NaN3); (b) Aerobically heat-treated in the presence of 10 mM NaN3; (c) Heat treated anaerobically.
As in the case with Candida, heat treatment under anaerobic conditions did not abolish the fluorescent SOSG staining (Fig. 4b) and addition of SOSG 24 h after the heat treatment resulted in fluorescent staining similar to that observed immediately after cooling the samples (Suppl. Fig. 7).
Similar results were obtained when SOSG fluorescent staining of S. aureus was examined (Suppl. Fig. 8).
SOSG has been used to demonstrate intracellular singlet oxygen production by photosensitizers38, by photosynthesis2, and by various stress factors in plants11,39,40. Irrespective of the increase of fluorescence intensity by irradiation of SOSG in the presence of photosensitizers, it has been reported that no reproducible results could be obtained by irradiation of SOSG containing cells9. To test the effect of photosensitized production of 1O2 on SOSG fluorescent staining of E. coli, we used Zn–porphyrin based photosensitizers with progressively increasing lipophilicity. We have previously reported that illumination of E. coli suspensions in the presence of amphiphilic Zn–porphyrins with six and eight carbon chains caused loss of membrane integrity, while hydrophilic 2–4 carbon chains analogs did not41. When E. coli cells suspensions were illuminated in the presence of Zn–porphyrin photosensitizers, only samples containing six and eight carbon chains photosensitizers displayed fluorescence (Suppl. Fig. 9). Only a small fraction of E. coli cells became fluorescent when illuminated with the four-carbon chain-containing ZnTnBu-3-PyP (Zn(II) meso-tetrakis(N–n-butylpyridinium-3-yl)porphyrin), and no fluorescence was observed in samples illuminated in the presence of Zn-porphyrins with shorter aliphatic chain (Suppl. Fig. 9). Similar results were observed when SOSG was used to detect 1O2 photo-generated by TMPyP (H2TM-4-PyP) (100 µM) and protoporphyrin IX (PpIX) (4 µM) concentration in a human nasopharyngeal carcinoma cell line CNE25. The planar hydrophilic metal-free porphyrin para ligand, like its metalated ZnTM-4-PyP analog32, intercalates in DNA and accumulates in the nucleus. The more lipophilic PpIX, like the amphiphilic Zn(II) meso-tetrakis(N-hexylpyridinium-3-yl) (ZnTnHex-3-PyP) and Zn(II) meso-tetrakis(N-octylpyridinium-3-yl) (ZnTnOct-3-PyP) porphyrins32, disperses predominantly in biomembranes, including plasma membrane. Upon illumination, SOSG fluorescence was observed only in carcinoma cells treated with PpIX, but not in cells treated with TMPyP5. Based on the impermeability of the plasma membrane for SOSG, the authors concluded that 1O2 generated by PpIX in the plasma membrane diffused out of the cell interior and interacted with SOSG in the extracellular environment. No explanation was provided for fluorescent staining of the whole cells but not of the medium on Fig. 3 (90 s) of the same publication5.
SOSG fluorescent staining of cancer cells
It has been hypothesized that incorporation of SOSG in mammalian cells is prevented by affinity binding of the fluorescent marker to components of the medium9 and staining of mammalian cells has been achieved only in modified medium. To prevent such binding, immediately before adding SOSG, the medium was replaced with PBS-glucose. For the relatively short time of incubation applied in this study, SOSG did not stain the cancer cells (Fig. 5a). The cells displayed bright green fluorescence only after permeabilization of the plasma membrane by heating (Fig. 5b), after treatment with chlorhexidine (Fig. 5c), or after treatment with tert-butyl hydroperoxide (Fig. 5d). Chlorhexidine and tert-butyl hydroperoxide, however, lysed substantial fraction of the cells.
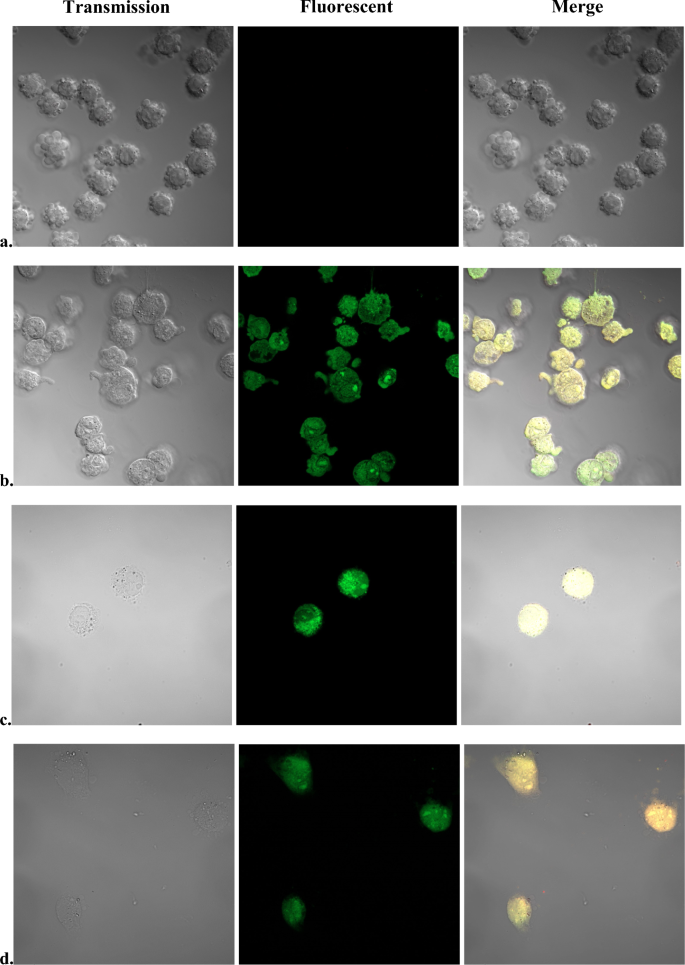
Fluorescent SOSG staining of pII cancer cells. Cells were grown in RPMI 1640 medium to a confluence of 70–90%. Immediately before treatment, growth medium was replaced with PBS-glucose. The cells were incubated 10 min either at 100 °C (b); at 25 °C with 10 µg/ml chlorhexidine (c); or with 3% tert-butyl hydroperoxide (d). Untreated controls (a) were kept at 25 °C during the duration of treatments.
Similar results were obtained when MCF-7 cancer cells were exposed to heat or to chlorhexidine (S. Fig. 10).
Fluorescent SOSG staining coinciding with loss of membrane integrity (propidium iodide staining) has been previously reported39, but has been attributed to generation of singlet oxygen.
This work did not investigate why SOSG became fluorescent when taken up by the cells. SOSG is made of two parts, anthracene and fluorescein, whose fluorescence is quenched by intramolecular electron transfer9. Reaction with singlet oxygen produces an endoperoxide which prevents the electron transfer and fluorescence is restored. Formation of endoperoxide, however, seems not to be the only mechanism preventing the electron transfer. Initial attempts to produce a singlet oxygen nanoprobe free from the pitfalls of SOSG resulted in a product with much higher fluorescence than SOSG42. The reason was SOSG microenvironment which prevented electron transfer, and as a result, quenching of the fluorescence was not efficient42. It is tempting to speculate that inside the cells, SOSG is exposed to natural agents which similar way suppress efficient electron transfer thus causing fluorescent staining of the cells even in the absence of singlet oxygen.